Literally Imagine Dragons
Crude oil is full of traces of a bygone world. One with different rules. Exotic chemistries. Fire-breathing dinosaurs.
Chlorophyll and heme—the green in plants and the red in blood, respectively—both belong to the same class of chemicals, the porphyrins. In fact, their core structures are nearly identical.
At a glance, the similarities are obvious; they share this beautiful dreamcatcher shape, an elaborate jewel setting for the metal atom at the center. Where heme holds an atom of iron, chlorophyll holds an atom of magnesium.
These are two of the most important chemicals on Earth.
Chlorophyll is the molecular antenna that makes photosynthesis possible. It’s the door through which nearly all chemical energy enters our biosphere. How many calories have you eaten in your lifetime that didn’t start out as sunlight at some point? And through that same process, spinning sugars out of thin air and sunlight, it helps produce the oxygen we breathe.
On the other side of the equation, hemoglobin lets us use that oxygen, carrying it in our blood cells until we turn it back into CO2.
One clear message is that this is a powerful, versatile chemical structure. That molecular ring enables some remarkable chemistry, in a remarkable diversity of ways.
In chlorophyll, the role of the central magnesium atom is somewhat passive: its job is to hold the ring together, pin it flat, and attach the whole thing in the right spot as part of a larger “antenna” protein. It’s the ring itself which does the work of receiving the sun’s energy and making useful work out of it, providing a conductive racetrack for electrons to zip around, accelerated by the corkscrewing electromagnetic wave of a photon.1
In heme, it’s the iron at the center which does the important work—gaining and losing electrons to grab or release oxygen atoms. Here, the ring is mostly a carrier. This reactivity is also why you’ll find heme at the active site of a huge variety of enzymes, in all branches of life. The cytochrome P450 enzymes,2 which help your body deal with toxins and metabolize drugs, all contain a heme molecule at their core.
It is, in many senses, the anvil on which chemical bonds are forged and broken.
There are other variations on the porphyrin theme, like vitamin B12. The gem at its center is cobalt—highly toxic, except in this elaborate containment apparatus, where we call it cobalamin.
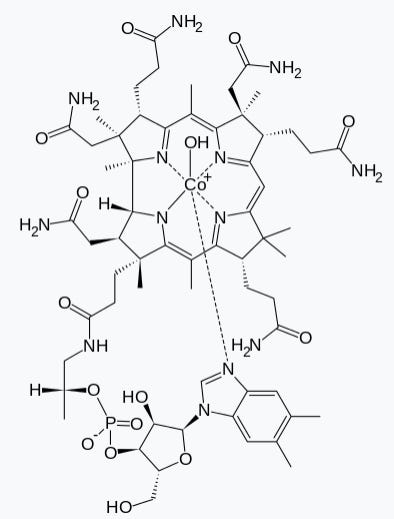
B12 is another “anvil”; practically any time you want to move a carbon atom from one molecule to another in your body, you’re using B12, albeit indirectly—it’s a key part of the folate cycle, essential for the synthesis of DNA.
In archaea, the weird third leg of the tree of life,3 porphyrins are key as well: one of the main roles of archaea in the biosphere is the production of methane, and the enzymes which enable that chemistry rely on hydrocorphin—a porphyrin with a nickel atom at its center. Methane flux plays a big role in shaping the climate by acting as a natural greenhouse gas, and in shaping the biosphere by feeding methanotrophic microbes.
So hopefully, by this point, you get it. Porphyrins and their derivatives are some of the strongest magic in biology. There are a few different types, each with different metal atoms at the center, which enable them to do a really interesting variety of things. Without them, life as we know it literally could not exist.
Also, just as importantly: they’re all pretty colors. Blood is red, plants are green, B12 is naturally a kind of hot pink, and the archaeal cofactor is yellow. They’re literally biological jewels.
I know not everyone else gets horn’t up about chemical structures the way I do, so I had MidJourney draw them up as actual jewels for you.
Ancient Power
So here’s the exciting part.
There are hints of a fifth. A lost porphyrin. The metal at its center? Vanadium. Named after a goddess of beauty, for its ability to take on any number of colors, depending on its oxidation state.
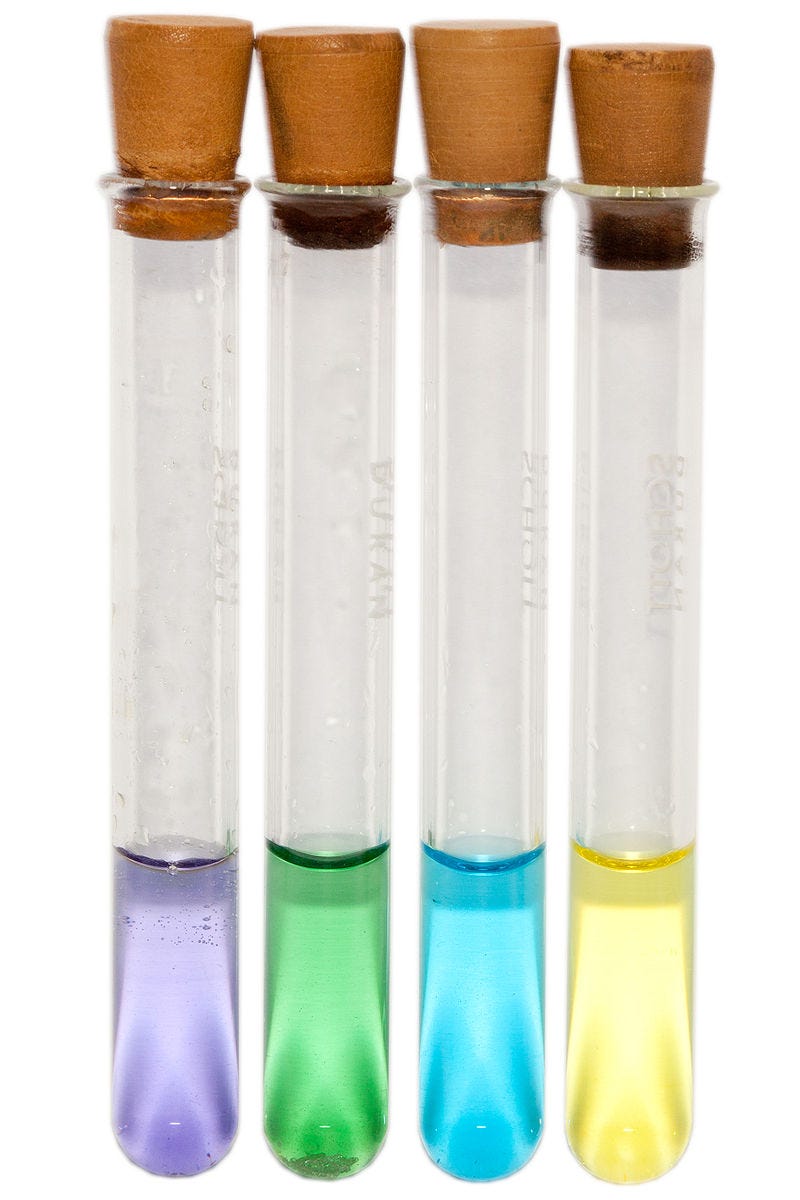
This vanadium-based porphyrin has only been found in crude oil, meaning it’s likely to have belonged to a life form that’s long extinct, or—if it still exists—is hidden.
As a result, we have no idea what the molecule was for. Consider: if you found a chlorophyll molecule on its own, completely without the plant for context, you’d never know that it gave its creator the incredible power to sip starlight. You need the whole ballet of molecular machinery, antenna proteins, the krebs cycle for that.
But think about the power of the four we know. These are processes that literally shape the world at a global scale. Photosynthesis. Methanogenesis. Oxygen-handling. One-carbon chemistry.
Fill in the blank.
Now, I’ve never seen an Avengers movie, but I know that, if you have four ancient and powerful jewels, each of which wields a unique magic that’s fundamental to life as we know it, and then you hear that—deep down in the black blood of the Earth—they’ve found a fifth?
It’s a good time to sit the fuck up and pay attention. What’s that? It seems to have been crafted by some ancient life-form that’s long since vanished beneath the sands of time, leaving this mysterious but clearly powerful artifact as practically the only trace?
Sick.
To anyone scrolling to the comment box to point out that “um, excuse me, certain species of fungi and sea squirts are known to accumulate vanadium…”—cut it, sea squirt. I read the wikipedia page on vanadium biochemistry too. This is different. This is a porphyrin, and there’s a lot of it—enough that it was one of the first big discoveries in petroleum chemistry, because oil from certain places has enough of the stuff to foul up a refinery. With something that abundant, if its catalytic function was anything close to the power of the other four it has huge implications for our understanding of ancient Earth’s biosphere.
Some Irresponsible Speculation
Okay. Do not believe anything I say for the rest of this post. Everything up ‘til here, you can take to the bank. What follows, I guess you could take to a cryptocurrency exchange, because I’m about to sell you a wild and fantastic dream with only the barest pretense of legitimacy.
With that said: let’s imagine what kind of chemistry vanadyl porphyrin might be for: something as powerful and useful as photosynthesis, or the ability to breathe oxygen.
Where would we even start?
So the periodic table is laid out the way it is because certain elements have similar properties. Sulfur and selenium are in the same column because they bond alike: most chemical compounds that contain a sulfur atom could have a selenium atom in its place instead. They even smell alike! Crack open a bottle of a supplement like methyl-seleno-methionine and you’ll be greeted by something a lot like the characteristic rotten-egg odor of brimstone. But there are also differences: selenium is a larger atom than sulfur, and can react in some ways that sulfur can’t.
The importance of these similarities (and differences) is on display in our biology: Selenium is an essential trace nutrient because the body has a select few proteins that require the selenium-substituted versions of sulfur-containing amino acids in order to function properly. Glutathione peroxidase, which helps control oxidative stress in the cell, is one—as are the enzymes that activate your thyroid hormones.
This similarity within columns of the table has led some to speculate that, since life as we know it here is carbon-based, and silicon is one row down from carbon in the periodic table, life on other planets might be based on silicon. It wouldn’t be a 1:1 substitution—the rules are different enough that you’d have whole other kinds of amino acids,4 but the general principle would be the same, because carbon and silicon both form stable, four-bond structures with hydrogen, oxygen, and other atoms of their same type. It’s an interesting thought experiment, because conditions where carbon-based life wouldn’t really work—like in the hydrocarbon seas of Titan—could still theoretically host some exotic form of life.
Cool as that is, it’s a little far-out for me to speculate on. But there’s a few cases where life here on Earth works with silicon, sort of like how it works with selenium—as an occasional sub-in for its lighter cousin, when it turns out that the differences in their chemical properties make it useful.
The stars of that show are diatoms: tiny phytoplankton that produce these incredibly complex and beautiful shells of glass for themselves by fusing silicon dissolved in ocean water. There’s a lot we still don’t understand about them, despite the fact that they’re responsible for about 20% of global carbon fixation, and have been for eons—blanketing the seafloor in layers so thick that we have a special name for a kind of rock made entirely of their desiccated shells. If I were going to pick an extant organism that’s likely to be hiding interesting new fundamental chemistry, these guys are near the top of the list. Maybe vanadium porphyrin is their secret.5
But where’s the fun in that? Let’s get imaginative.
Think about methanogenesis, the process where microbes turn hydrogen (H2) and carbon dioxide (CO2) into methane (CH4). The microorganisms that do this, methanogens, are important auxiliary players in the microbiomes of cows and other ruminants. This is why you sometimes see videos of people flaring off the methane from a bloaty cow like it’s a natural gas well that’s blown a leak.
See, the bacteria that help cows break down fiber for energy produce a lot of hydrogen in the process. If that hydrogen doesn’t have somewhere to go, the buildup slows down the fermentation process, making it less efficient. Methanogens earn their keep in a cow’s gut by taking the hydrogen from that environment and reacting it with carbon dioxide to turn it into methane, which doesn’t inhibit fermentation in the same way. This lets the animal burp less, and get more calories from its food.
So let’s imagine some ancient microbe that, armed with its vanadium porphyrin, possessed a parallel power, one step down the periodic table: Silanogenesis, turning hydrogen and SiO2 into silane: SiH4. Simple enough, right?
Silicon dioxide, SiO2, is silica: sand, quartz, stone. It’s the most abundant mineral on Earth, and it’s not at all like its carbon cousin CO2—at least at temperatures we’re used to. But silane, SiH4, behaves a lot like its carbon cousin methane. At room temperature, it’s a flammable gas.
So all I’m saying here is that, if silanogenesis were biologically possible via some lost chemistry like a vanadium porphyrin, there’d be a really interesting niche for a microorganism that does it. Such an organism, as part of a microbial community, could fit into basically the same ecological niche that methanogens occupy in the ruminants of today—scrubbing out hydrogen produced via fermentation by reacting it into silane. All they’d need is SiO2 in order for that to work, and there’s enough of that around…although you wouldn’t expect to find much of it in an animal’s gut, unless they were eating rocks.
Oh, wait. Don’t they sometimes find piles of smooth-polished rocks, nestled neatly in the fossilized ribcages of herbivorous dinosaurs?
As a matter of fact, they do.
But didn’t dinosaurs just eat those rocks to facilitate a grinding, milling action in the gut, a sort of secondary chewing process?
Well…apparently, no. They’ve observed a similar process in modern birds, but neither the mass or the texture of dinosaurs’ stomach-stones is consistent with that interpretation.
Huh! A mystery, then. Surely, it’s just an interesting coincidence that these stones would provide a convenient source of mineral silicates.
But imagine it’s not.
Imagine that, back a billion years ago, some prehistoric microbe figured out how to get energy by turning rocks and hydrogen into silane, and bloomed—thriving so vigorously that its descendants spanned the globe, becoming part of the enormous mats of biological material that would eventually be pressed into the ocean floor, cooked by time and geology into petroleum, leaving its vanadium anvil as the only recognizable trace.
Imagine that, just like methanogens, some of this wild microbe’s descendants found refuge in the GI tract of a vertebrate, earning their keep by facilitating fermentation, reducing the partial pressure of hydrogen in the gut by reacting it into silane.
It follows logically that, just like in modern ruminant, this gas would have to be periodically expelled, likely as a belch.
It’s not that much of a stretch to imagine a world where dinosaurs burped out clouds of silane, is all I’m saying.
And I can hear you asking: Why? Why go to all this trouble for stegosaurus burps?
Because, dear reader, I have kept something important from you.
Silane is not just flammable. It is what we call pyrophoric.
It spontaneously ignites in the presence of oxygen.
Part of the reason I was tickled enough by the idea to write this piece is that a pyrophoric gas produced by fermentation in the GI tract would resolve some of the biggest difficulties with having a fire-breathing animal, namely: how do you manage ignition, and how do you avoid burning your own face off?
Pyrophoricity solves both of these in one swoop; a long breath-hold yields a lungful of exhaled CO2, which could help to get a burped-up cloud of silane out of the airway and into your predator’s face before reaching a fuel-to-air ratio suitable for spontaneous combustion.
Now, like I said before, all of this is just wild speculation in the interest of fun. Some geologists think vanadium porphyrin is just chlorophyll that’s had its magnesium displaced, and there’s no real reason to connect vanadium porphyrin to this hypothetical metabolism.
But the other reason this idea has stuck with me is that it’s strikingly plausible from the perspectives of evolutionary complexity and incentive alignment. Sure, you could just say “a fire-breathing dinosaur would be fitter than a non-fire-breathing one, so they probably breathed fire”, but that’s cheating—evolution has to happen one step at a time, and each step needs to work on its own.
This is why a fire-breathing T-rex doesn’t work: they didn’t eat plants, and had no need of hydrogen scrubbing in their guts. But with silanogenesis in an herbivorous dinosaur, we’ve stumbled on a process that already works well enough at the micro level to be totally plausible, for the same reasons methanogenesis occurs in the ruminants of today. The fact that it would give its host a truly remarkable defense against predators? That’s just gravy: the kind of emergent benefit that you’d really only expect to see arise secondary to a more basic alignment of interests, but which—when it shows up—can give a creature a huge evolutionary edge.
I will draw the line at positing this as an explanation for the prevalence of fire-breathing dragon myths. That’s a bridge too far. After all, how would we even have come to know of their existence, aside from some kind of subconscious ancestral memory from before the extinction of the dinosaurs?
We are descended from creatures that lived through that age, though. If it did happen, some early mammal would have seen it. And although simple memories like “[odor x] = pain” can be encoded by one generation and recovered by the next (which is e.g. why everyone instinctively hates the smell of burning hair), I’m hard-pressed to imagine a concept as complex as “fire breathing lizard” making it through intact.
Although, if any animal could do it, it’d be humans. And if you asked me to imagine how you’d go about it, dredging up a full image memory from the tar pits of our prehistoric past…well, I’d probably tell you to get a bunch of humans together and have them make up stories, and tell them to each other, and elaborate on one another’s tales. And maybe, in the pool of their collective unconscious, certain things would resonate, for reasons nobody could fully explain.
All I’m saying is…
—🖖🏼💩
I am (as one always must with quantum physics metaphors) lying a little bit about how this works. But only a little!
Of “Grapefruit juice and valium” fame
The two main legs are prokaryotes and eukaryotes. To those who would sputter “b-b-but trees don’t have legs!”, I say: relax, it’s an analogy. Also: Treebeard.
Or would they be phosphono-acids? An arsenate backbone to the DNA?
Biochemistry is funny. It’s extremely easy to get a yes/no answer to questions of the form “Does substance X contain chemical Y?” by running it through a mass spec, but any biological matrix contains so much random stuff that to ask “What chemicals does substance X contain?” will leave you buried beneath an onslaught of unknown ion peaks that you could never possibly hope to annotate.
Thank you. I am always entertained and find something thought provoking. I also wonder, at times, if you teleport in to my mind space. This time because I have spent days and weeks and months over the past 8 years looking at tetrapyrroles/porphyrins and implications in Parkinson’s since we found elevated aminolevulenic acid levels in some of John’s tests and we found signal in genes involved in photosynthesis- phytoene desaturase. It made me dig deeply in to photosynthesis, purple photosynthetic bacteria, and the production of sugars in the gut. While I can say at this point I have no answers I do believe that heme molecules are key to many processes and the pollution of our food and environment are having a significant impact on this. See hydrogen sulfide and methane producing bacteria and archaea in PD gut research. 😉🤔
This is great. Interesting, amusing, and whimsical. Thank you for it!